It’s been a while since I last wrote on my blog, and in that time, I had an epiphany. Here I am learning about the environment, but most of my blog has been about technology. I’m not even an engineering major! So, guess what, I’m writing about water treatment in river restoration. I had a blast going to the Kallang River restoration project at Bishan-Ang Mo Kio Park on 27 February and having my eyes opened to what my blog has been missing out on. Plants! Animals! Biodiversity! Continue reading
Category Archives: Uncategorized
A Murky Future
The end of the semester is coming up, and as I draw my blog to a close, I suppose it is fitting to discuss the cutting-edge of water treatment techniques in Singapore.

Innovation in Water Singapore Volume 11 by PUB. Source: https://www.pub.gov.sg/Documents/Issue%2011_Innovation%20in%20Water,%20Singapore_Full%20PDF.pdf
Innovation in Water Singapore is a yearly publication by PUB that highlights research and developments in water treatment technology that I would recommend you check out!
The 2018 and 2019 issues both featured algal blooming as a subject of concern and for good reason. Even without considering excessive nutrient loading from industries, a warming urban environment (Roth & Chow, 2012) still makes freshwater bodies an inviting place for algae to proliferate (Lai, Chua, Chan, 2018). One of our national taps source water from these water bodies; and the presence of algal blooms clog up NEWater’s signature reverse osmosis (RO) membranes, adding to RO’s already high energy usage and membrane maintenance (Ooi et al., 2019). I don’t think murky green waterways littered with dead fish (Ooi et al., 2019) would be very appealing, wouldn’t you agree?

Algal blooming at Kraji Reservoir. Photo by an ST reader Source: https://www.straitstimes.com/singapore/environment/algae-at-kranji-reservoir-turns-waters-a-murky-green-pub-says-no-cause-for
In our discussions of eutrophication, treatment measures for algal blooms mostly revolved around reductions in nutrient loading. Alternatively, the 2018 issue showcased ultrasonic disinfection, where a pilot study was conducted on the Serangoon Reservoir (Lai et al., 2018). Its principle of operation is the exciting part: where cavitation bubbles form and implode, creating an environment where water decomposes and forms biocidal hydroxyl radicals that eliminate algal cells (Joyce, Wu, & Mason, 2010).
Operating like a floating fish tank filter, closed water systems like a catchment could conceivably be protected from algal blooms (Lai et al, 2018). Conversely, it would be a lost cause in open waters where desalination plants draw seawater from. Ultimately, the best solution lies in managing the growth factors of algae: nutrients and warmth. That would mean dealing with warming seas, urban heating, and anthropogenic nutrient inputs: each is a tall order in their own right.
Concerning last week’s post, PUB’s 2017 issue indicates that they already dabbled into Membrane Contactor technology! If you have not read last week’s post, Membrane Contactors are one of many liquid-phase extraction technologies, which also encompasses RO. In particular, Membrane Contactors offer opportunities for resource recovery: in this case, the recovery of methane from anaerobic bioreactors (Wang, Bae, 2017). Unlike VOC recovery which features a liquid extractor, either the property of pressure gradient force on gases is exploited by using a vacuum, or carrier gas(es) with high affinity for biogas are utilized.
This adds on to Ryan’s comment about political will for the implementation of such technologies in Singapore. The realisation hit me that there is a strong political will for the implementation of novel and critical technologies in wastewater treatment; just not in the places that need it the most! If this project tenure was awarded to point-source emitters of high-VOC wastewaters, widespread industrial adoption of wastewater resource-recovery and treatment technologies could have begun years ago!
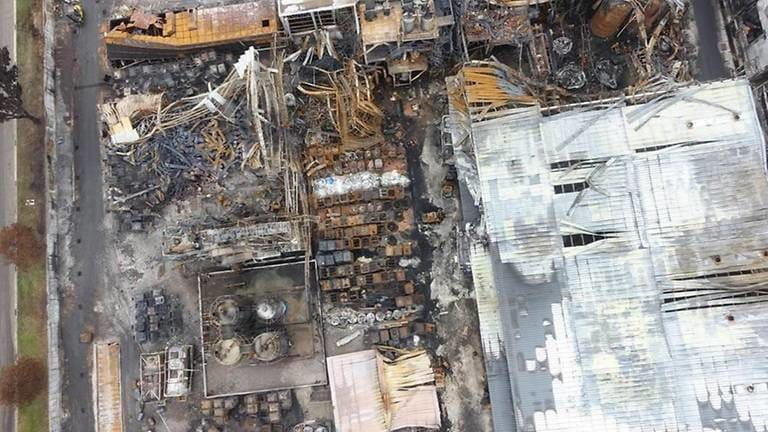
Could the 2017 ECOSWM fire have been avoided with PUB’s research involvement? Source: https://www.channelnewsasia.com/news/singapore/tuas-plant-fire-eco-special-waste-management-fined-mom-11848164
While it is invigorating to know that such technological advancements are taking place, the reach of Singapore’s political will is not yet enough to provide holistic solutions to our water problems. We already have the research, now its time to implement them where they are needed.
References
Joyce, E. M., Wu, X., & Mason, T. J. (2010). Effect of ultrasonic frequency and power on algae suspensions. Journal of Environmental Science and Health – Part A Toxic/Hazardous Substances and Environmental Engineering. https://doi.org/10.1080/10934521003709065
Lai C. L., Chua C. Y. , Chan W. S. (2018). Managing microalgae growth with ultra-low frequency technology. Innovation in Water Singapore, Vol 10, 27. Retrieved from https://www.pub.gov.sg/Documents/Innovation_in_Water_Singapore_Vol10.pdf
Ooi S. K., Babovic V., Navarro L. M., Yim S. S. P., Wang M. Y. | NUS; Xia L. J., Gao R. M., Sim M., Mahadevan A. | PUB;
Tay S. H. X. | H2i (formerly NUS) (2019). An algal proliferation prediction system for Singapore’s coastal waters. Innovation in Water Singapore, Vol 11, 40. Retrieved from https://www.pub.gov.sg/Documents/Issue%2011_Innovation%20in%20Water,%20Singapore_Full%20PDF.pdf
Roth, M., & Chow, W. T. L. (2012). A historical review and assessment of urban heat island research in Singapore. Singapore Journal of Tropical Geography. https://doi.org/10.1111/sjtg.12003
Wang R., Bae T. H., Y. Zhang (2017). Maximising biogas recovery for used water treatment. Innovation in Water Singapore, Vol 9, 27. Retrieved from https://www.pub.gov.sg/Documents/PUB_InnovationinWater_Issue9.pdf
The Fiery Underbelly of Singapore
From mutant superbugs to foul-smelling but helpful microbes, this week’s post will cover a truly blistering affair right in our own backyards that you might not have heard of!
Singapore’s water management policies and clean water supply seem to indicate that our water situation is fine and dandy. However, you might be surprised to learn that the unsafe release of toxic or volatile organic compounds (VOCs) into Singapore’s wastewater streams are a recurring and unfortunately common occurrence if these news articles[1][2][3] are any indication.
Among the offenders are licensed toxic industrial waste collectors (TIWCs) like NSL Oilchem and Cramoil that handle almost any hazardous waste under the sun, or at least they are supposed to. But before we point any fingers, consider this analogy: you are given a stew and told that you must extract every last drop of water out of it using your household equipment without burning or damaging any of the ingredients, or face a fine. Now imagine that some of the ingredients cannot be removed and can spontaneously combust!
This is the predicament that many TIWCs are in: dealing with highly varied liquors of various VOCs from industries. Many of these VOCs like alcohols, phenols and polyaromatic hydrocarbons seem impervious to municipal wastewater treatment technologies (Paxéus, 1996), requiring specialised equipment that these TIWCs have. Even so, once TIWCs have isolated highly concentrated liquors of VOCs from industrial wastewater, they have to find ways to store a highly flammable and varied VOC mixture with poor industrial reusability before disposal by incineration.
That is just an accident waiting to happen! Case and point, the 2017 ECO Special Waste Management fire was caused by mishandling of extracted hexane and dimethyl benzene (Xylenes) from industrial wastewater[4].

2017 ECO Special Waste Management facility on fire. (Photo by Mohamad Danial) Source: https://www.straitstimes.com/singapore/cause-of-tuas-plant-blaze-under-investigation
Instead, if we shift the onus of VOC wastewater to the point-source emitters: the industries in Singapore, this essentially kills two birds with one stone! Industries can recover isolated volatile reagents from their own wastewater and immediately reuse them without risky storage and handling; there is less wastewater sludge ash we have to landfill.

Burnt liquid hexane storage tanks, illustrating the risks of storage and handling VOCs. (Photo by Ministry of Manpower) Source: https://www.channelnewsasia.com/news/singapore/tuas-plant-fire-eco-special-waste-management-fined-mom-11848164
The proverbial silver bullet technology is a Membrane Contactor. It utilises liquid extraction principles (Anil Kumar Pabby, Syed S.H. Rizvi, 2015; B.W. Reed, M.J. Semmens, 1995) where a liquid extractant designed to have a high affinity to the desired product (VOC) draws the chemical away from wastewater through a porous hydrophobic membrane by diffusion. The extracted product can then be isolated from the designer extractant, and both the extractant and the product can be reused.
Sounds too good to be true? Industrial-grade membrane contactors are already on the market, like 3M’s Liqui-CelTM range here:
There are even cases industrial applications for the recovery of industrial reagents from wastewater (Anil Kumar Pabby, Syed S.H. Rizvi, 2015)! If KoSa Netherlands BV can find economic viability with such a system, I believe industries in Singapore should follow their lead. All it takes is for industries and the government to get their minds off maximum profits and economic growth, and think a little more of safety and environmental sustainability.
References:
Anil Kumar Pabby, Syed S.H. Rizvi, A. M. S. R. (2015). Handbook of Membrane Separations Chemical, Pharmaceutical, Food, and Biotechnological Applications (Second Edi). Taylor & Francis. ISBN 9781466555563
B.W. Reed, M.J. Semmens, E. L. C. (1995). Membrane Contactors. In S. A. S. R.D. Noble (Ed.), Membrane Separations Technology: Principles and Applications (pp. 467–496). Elsevier. ISBN 9780444816337
Paxéus, N. (1996). Organic pollutants in the effluents of large wastewater treatment plants in Sweden. Water Research. https://doi.org/10.1016/0043-1354(95)00278-2
[1] PUB media release on Cramoil repeat offence in 2018 for discharging hazardous VOCs – http://www.nas.gov.sg/archivesonline/data/pdfdoc/20180425010/PUB%20ISSUES%20STOP%20ORDER%20ON%20CRAMOIL%20SINGAPORE%20PTE%20LTD_press%20release%2025%20Apr%202018.pdf
[2] 38 firms charged with releasing dangerous substances and VOC in 2019 – https://www.straitstimes.com/singapore/health/illegal-discharge-into-sewers-38-firms-punished
[3] 5 firms charged with releasing prohibited VOC and heavy metals in 2018 – https://www.straitstimes.com/singapore/five-firms-fined-for-illegally-discharging-waste-into-public-sewers
[4] https://www.channelnewsasia.com/news/singapore/tuas-plant-fire-eco-special-waste-management-fined-mom-11848164
Smelly Self-Sufficiency?
About two weeks ago, we paid a visit to our campus’ own BCA Green Mark (Platinum) certified (BCA, 2018) net-zero energy building at SDE4. While SDE4’s energy-saving, energy-producing, and innovative construction measures were intriguing, what got my water-obsessed brain fired up were its water recycling facilities.
Under BCA’s Green Mark Criteria (BCA, 2016), points are awarded where non-potable water scavenged is used to reduce potable water usage. SDE4 achieves this using a rainwater harvesting system, where two-thirds is used for flushing and irrigation of gardens, and the remaining is fed through a simulated wetland called a bio-retention basin before being discharged into sewers, as seen below.
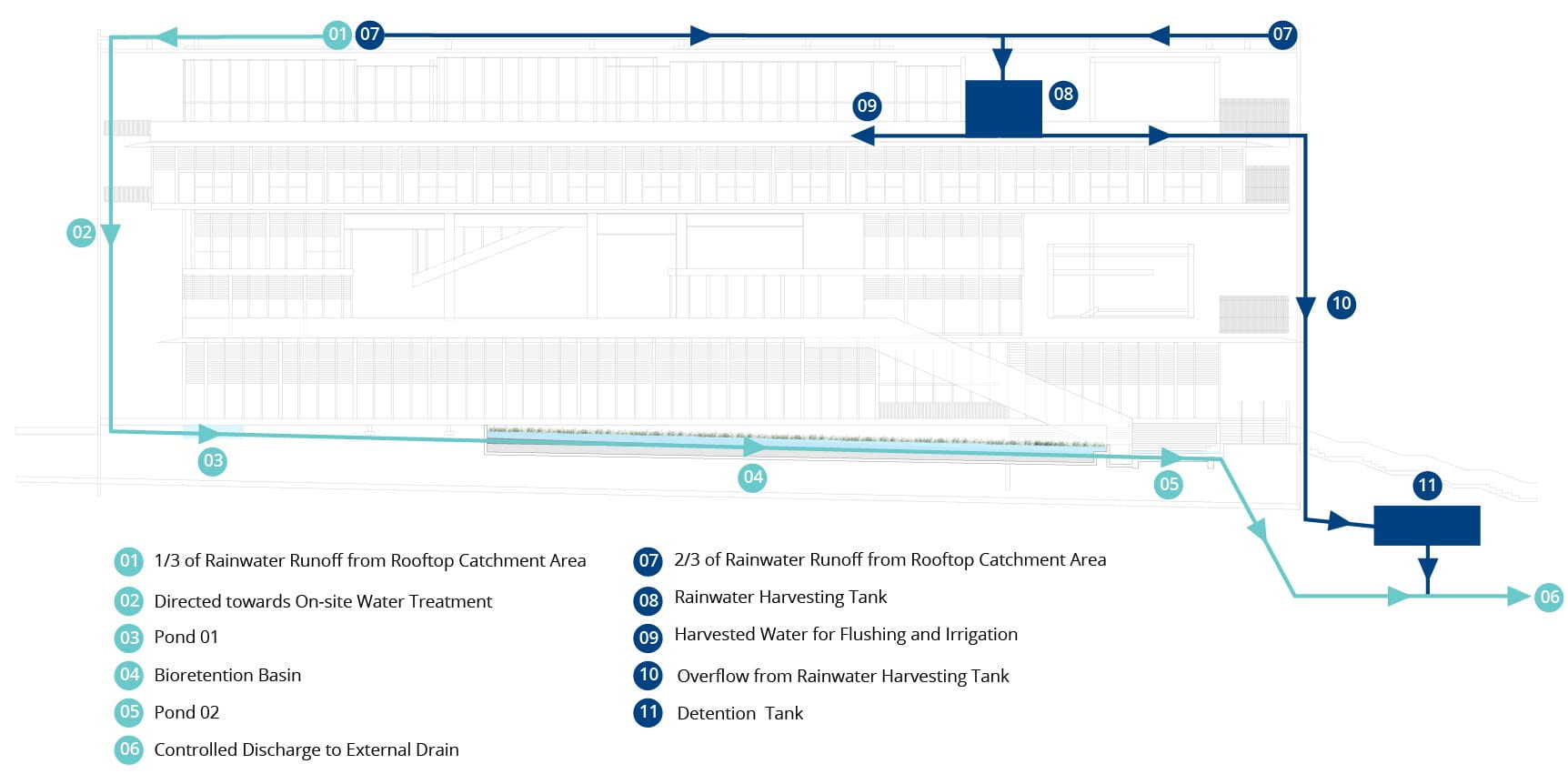
Schematic of SDE4’s rainwater harvesting and recycling system. Source: https://www.learningtrail.io/WATER_TRAIL/WATER_TRAIL

Diagram of SDE4’s Bio-retention Basin. Source: https://www.learningtrail.io/WATER_TRAIL/BIO_RETENTION_BASIN

The Bio-retention Basin has a similar design to a Vertical Flow Wetland that is used for sewage treatment. Its abilities include reducing high BOD, suspended solids and pathogenic loads, and converting ammonia to nitrogenous oxides by wetland microbes. (Tilley, Lüthi, Morel, Zurbrügg, & Schertenleib, 2008). Source: http://www.iwa-network.org/wp-content/uploads/2016/06/Compendium-Sanitation-Systems-and-Technologies.pdf
This essentially makes SDE4 one of our 4 national taps: a mini catchment area!
While walking around the learning trails, something under the sink outside the fifth-floor design studios caught my eye.

A small biological treatment tank I found outside the Design Studio.
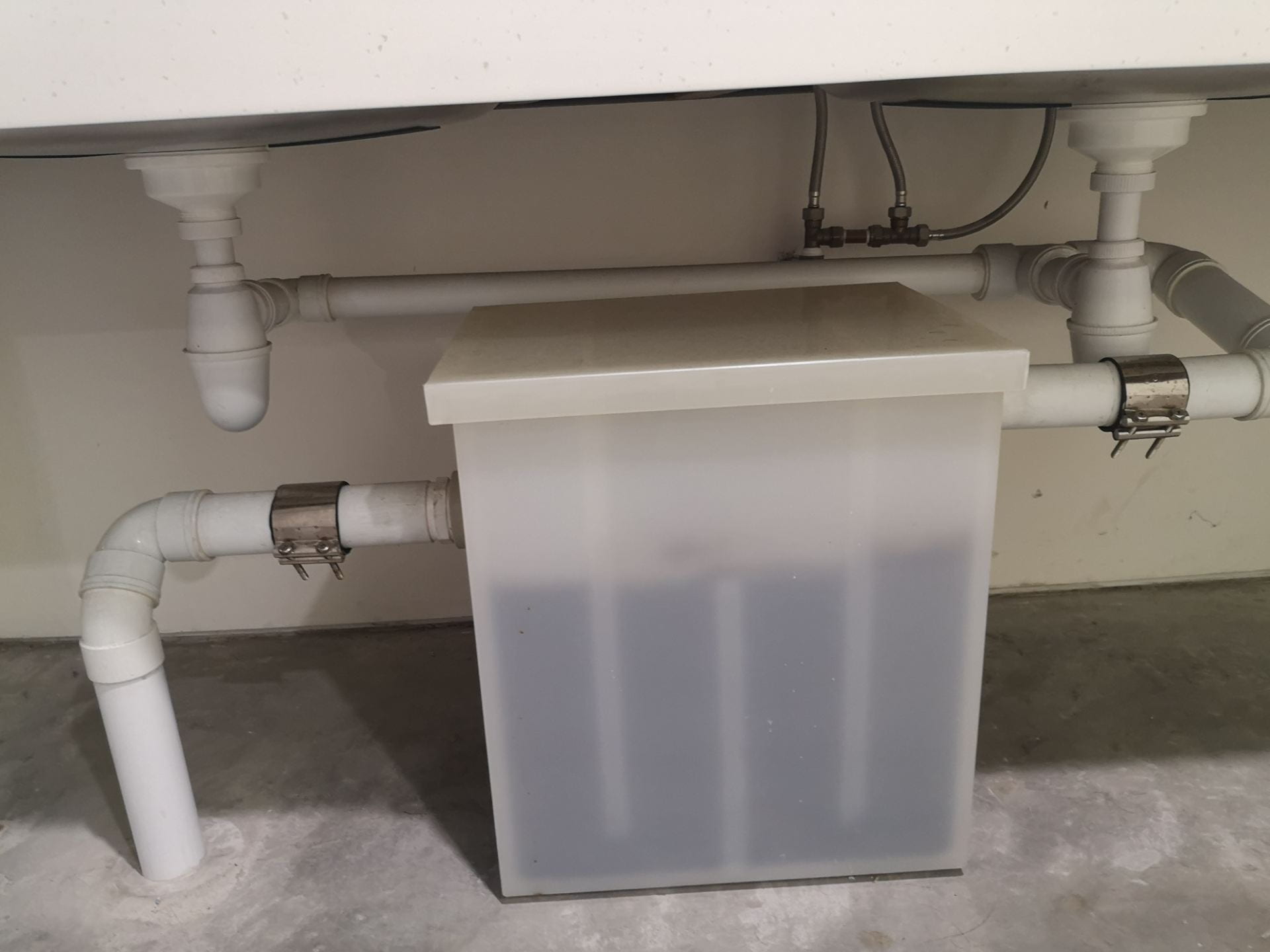
Alternative baffles can be seen, giving wastewater pollutants a higher retention time inside the reactor for the bacteria do decompose the pollutants.
Since that was the first bioreactor I have seen in person, I couldn’t help but open it.

There are visible improvements in water clarity and amount of scum produced as the water proceeds through the bio-reactor.
As vile as it looks, the smell was not particularly objectionable and similar to a mangrove swamp, hinting its modus operandi.
From what I can surmise, the reactor design incorporates elements from an anaerobic baffled bioreactor as pictured below.
Exposure to aerobic conditions above the surface and anoxic conditions below the surface might increase the diversity of the microbial community in the reactor, thereby expanding the range of pollutants it can handle. This might be appropriate since Design Studio personnel handle resins and aerosol solvents that are prohibited under the SDA effluent discharge limits. Unfortunately, I couldn’t get clarification from the SDE on this instalment.
With the building’s energy budget as constrained as it is, this bioreactor fits right in since the bacteria do all the work without any extra energy (Tilley et al., 2008)! However, without any sort of disinfection system or a clarification tank to remove any remaining pathogens and microbes, recycling the resulting effluent might not be so wise (Tilley et al., 2008).
Hence, I decided to look for low-energy alternatives for water recycling solutions; and what I found was a two-for-one deal!
Several studies have investigated the applicability of incinerator fly ash and bottom ash as an adsorbent for wastewater pollutants. Conventionally, this is done using activated carbon, which is costly (Ahmaruzzaman & Gupta, 2011). Conversely, fly and bottom ash are produced by the truckload every day. They have proven themselves against a wide variety of simulated wastewaters including pharmaceuticals & petrochemicals (Sunil J. Kulkarni, Sonali R. Dhokpande, 2013) and dyes (Gupta et al., 2005) which may be produced by the Design Studio personnel. The resulting effluent could contribute to the non-potable water demands of the building.
These techniques have not been rigorously tested and applied industrially, unlike energy-intensive reverse osmosis or activated carbon (Ahmaruzzaman & Gupta, 2011). However, SDE4 provides an amazing platform for pilot scale fixtures to be tested such that they may be feasibly applied to other buildings to spur low-energy water recycling and self-sufficiency!
References:
Ahmaruzzaman, M., & Gupta, V. K. (2011). Rice husk and its ash as low-cost adsorbents in water and wastewater treatment. Industrial and Engineering Chemistry Research. https://doi.org/10.1021/ie201477c
BCA. (2016). GREEN MARK FOR NON-RESIDENTIAL BUILDINGS NRB: 2015 including Hawker Centres, Healthcare Facilities, Laboratory Buildings and Schools. Building and Construction Authority Green Mark, 73. Retrieved from https://www.bca.gov.sg/GreenMark/others/Green_Mark_NRB_2015_Criteria.pdf
BCA. (2018). BCA Awards 2018. Building and Construction Authority Green Mark, 85. Retrieved from https://www.bca.gov.sg/GreenMark/others/gm2018.pdf
Gupta, V. K., Ali, I., Saini, V. K., Van Gerven, T., Van Bruggen, B. Der, & Vandecasteele, C. (2005). Removal of dyes from wastewater using bottom ash. Industrial and Engineering Chemistry Research. https://doi.org/10.1021/ie0500220
Sunil J. Kulkarni, Sonali R. Dhokpande, J. P. K. (2013). Studies On Flyash As An Adsorbent For Removal Of Various Pollutants From Wastewater. International Journal of Engineering Research & Technology, 2(5), 1190–1195. Retrieved from https://www.ijert.org/research/studies-on-flyash-as-an-adsorbent-for-removal-of-various-pollutants-from-wastewater-IJERTV2IS50668.pdf
Tilley, E., Lüthi, C., Morel, A., Zurbrügg, C., & Schertenleib, R. (2008). Compendium of Sanitation Systems and Technologies. In Development. Retrieved from http://www.iwa-network.org/wp-content/uploads/2016/06/Compendium-Sanitation-Systems-and-Technologies.pdf
Phosphorus: Our Other Black Gold
It is quite literally one of the few elements that hold us together. Without it, almost every oxygen-respiring organism would slump into an organic soup (Ruttenberg, 2013) More importantly, it supplies energy to most aerobic organisms’ cells, allowing lifeless organic compounds to move, grow and well, be alive (Ruttenberg, 2013)!

The effects of phosphorus fertiliser use on crops. Source: Franklin D. Roosevelt Presidential Library and Museum (53227(1828)
In agriculture, phosphorus is a vital ingredient in fertilisers that prevent topsoil degradation after harvests have removed soil nutrients (Cordell, Drangert, & White, 2009). This phosphorus is mined from rocks (aptly named apatite) that have substantial concentrations of phosphorus (Daneshgar, Callegari, Capodaglio, & Vaccari, 2018), supplied by tectonic uplift of buried oceanic sediments as seen below (Ruttenberg, 2013).

The global phosphorus cycle. Upward arrows indicate phosphorus replenishment. Source: Ruttenberg et al. (2013)
Our society effectively relies on a resource that takes tens of thousands of years to replenish to feed an ever-growing population (Cordell et al., 2009; Ruttenberg, 2013)! Unsurprisingly, we exploit this finite resource like there’s no tomorrow (Daneshgar et al., 2018). We pump more phosphorus than the ground can hold, causing the excess to leach into our rivers and oceans; stimulating toxic algae blooms that kill fish and create anoxic dead zones when the nutrients have been exhausted (MIT, 2016).
The depletion of phosphorus reserves is a hotly debated topic (Daneshgar et al., 2018). Daneshgar et al. (2018) gave a tentative estimate of a few centuries, while Cordell et al. (2009) painted a grim few decades remaining of unequal phosphorus distribution between the rich and poor. As an environmentalist, the concept of relying on a finite resource gives me the same anxiety as a looming deadline; it’s an itch that I cannot tolerate.

Anthropogenic phosphorus budget, landfill and sewage losses are fairly significant. Source: Cordell et al. (2009)
Ultimately, the ideal outcome is closing the anthropogenic phosphorus loop and preserving natural phosphorus flows. The recovery of phosphorus from wastewater treatment, while not a large proportion of anthropogenic phosphorus loss, is still a significant point-source emitter that we can feasibly amend (Cordell et al., 2009; Daneshgar et al., 2018). Furthermore, the inherent global distribution of wastewater treatment plants (WWTP) could equalise the current oligopoly of phosphorus source countries (Daneshgar et al., 2018). At least, it’s better than twiddling our thumbs.

Source: PUB
In conventional WWTPs, most of the phosphorus in wastewater is removed in sludge to meet effluent discharge standards and lost when the sludge is incinerated and landfilled (Cornel & Schaum, 2009).
Phosphorus-recovering WWTPs typically utilise magnesium and calcium salts to crystallise phosphate fertilisers from wastewater, wet sludge or incinerated sludge ash after pre-treatment to remove other pollutants like heavy metals (Cornel & Schaum, 2009). Other novel approaches such as the use of algae sequestration have been tested, but trade away phosphorus recovery performance for the production of ready-made livestock feed and organic fertiliser (Shilton, Powell, & Guieysse, 2012).
In the pursuit of recovering and reusing a finite resource, we should not lose sight of other environmental objectives too. After all, it would be pointless if phosphorus recovery uses more energy than phosphorus extraction from apatite (Daneshgar et al., 2018) since we would be exchanging different forms of environmental degradation.
Just imagine if our upcoming Integrated Waste Management Facility had a zero-energy phosphorus recovery system; we could be one step closer to sustainable food self-sufficiency!
References:
Cordell, D., Drangert, J. O., & White, S. (2009). The story of phosphorus: Global food security and food for thought. Global Environmental Change. https://doi.org/10.1016/j.gloenvcha.2008.10.009
Cornel, P., & Schaum, C. (2009). Phosphorus recovery from wastewater: Needs, technologies and costs. Water Science and Technology. https://doi.org/10.2166/wst.2009.045
Daneshgar, S., Callegari, A., Capodaglio, A. G., & Vaccari, D. (2018). The potential phosphorus crisis: Resource conservation and possible escape technologies: A review. Resources. https://doi.org/10.3390/resources7020037
Massachusetts Institute of Technology(MIT) (2016). Eliminating depletion and environmental damage with efficient phosphorus use and reuse. Mission 2016. Retrieved from https://web.mit.edu/12.000/www/m2016/finalwebsite/solutions/phosphorus.html
Ruttenberg, K. C. (2013). The Global Phosphorus Cycle. In Treatise on Geochemistry: Second Edition. https://doi.org/10.1016/B978-0-08-095975-7.00813-5
Shilton, A. N., Powell, N., & Guieysse, B. (2012). Plant based phosphorus recovery from wastewater via algae and macrophytes. Current Opinion in Biotechnology. https://doi.org/10.1016/j.copbio.2012.07.002
End of the Line
So, you’ve finished your apple and are left with its core, and you do not have access to a composter; what would you do?
Throw it in the bin, I hope! From there, our trash ends up at one of four Waste-to-Energy incinerators, where the majority of its water content and bulk is reduced to bottom ash† (BA) to save space. This BA is then transferred together with sludge ash from PUB’s wastewater treatment plants (WWTP) (PUB, 2016) and Toxic Industrial Waste Collectors* by barge to Pulau Semakau, where it is dumped into the sea.
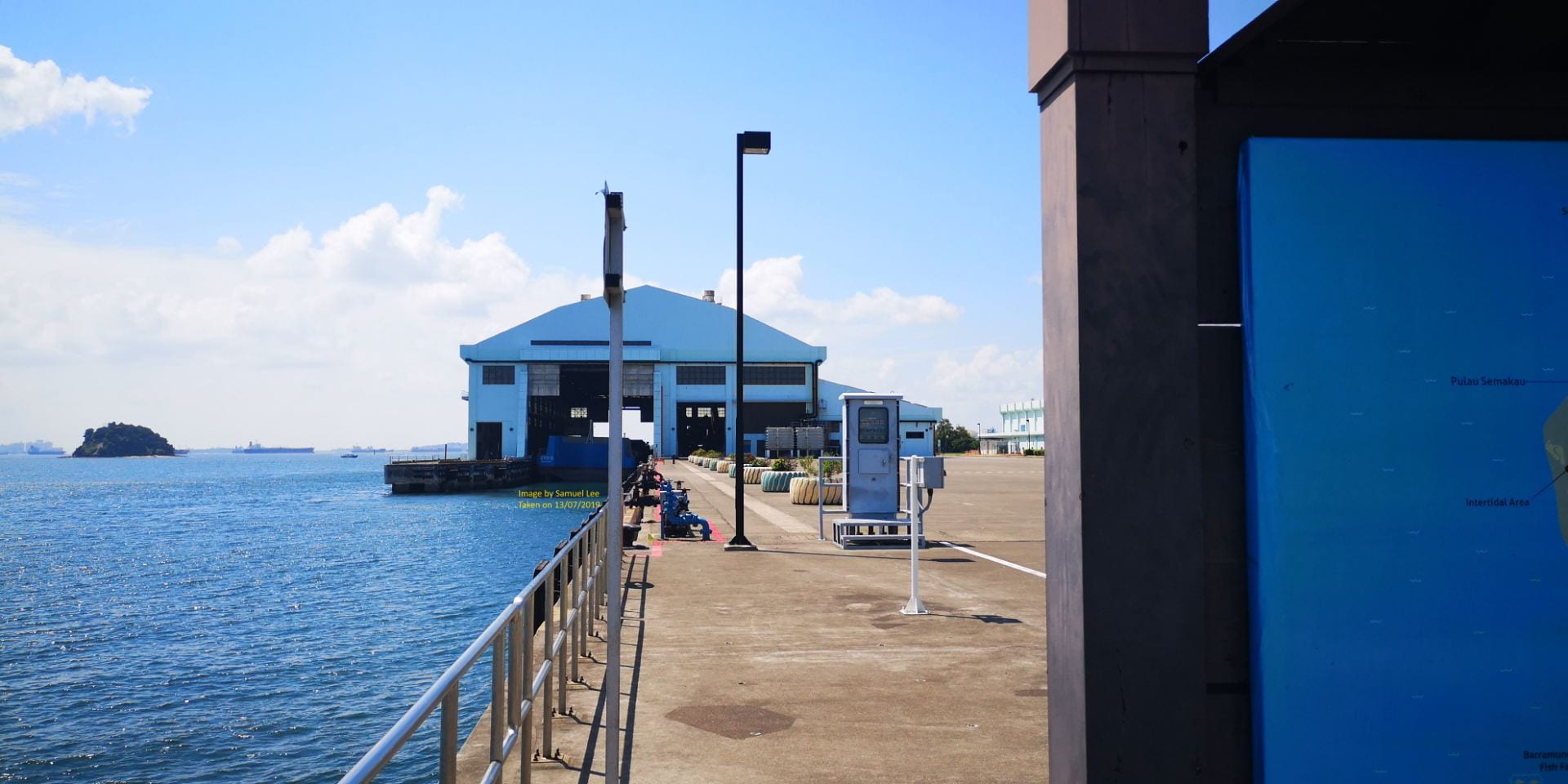
Bottom ash is transferred by barge to this transfer station
But wait, would that mean that hazardous substances from toxic industrial ash could perhaps leach into the sea? This could very well be the case. BA usually contains heavy metals(Lin, Wu, & Liu, 2007) and refractory halide-containing organic compounds, possibly from BOCs in WWTP sludge (Dung, Vassilieva, Swennen, & Cappuyns, 2018). Needless to say, these pollutants really should not be leaching into the sea due to their mutagenic or biocidal effects(Manaia et al., 2018; Tchounwou, Yedjou, Patlolla, & Sutton, 2012). Their persistence to biodegradation causes them to bio-accumulate and bio-magnify in apex predators and eventually us.
With these concerns in mind, I had the privilege of going to Pulau Semakau twice to find out how Singapore deals with the BA leachate issue. What I found clarified my woes, but raised other questions too.
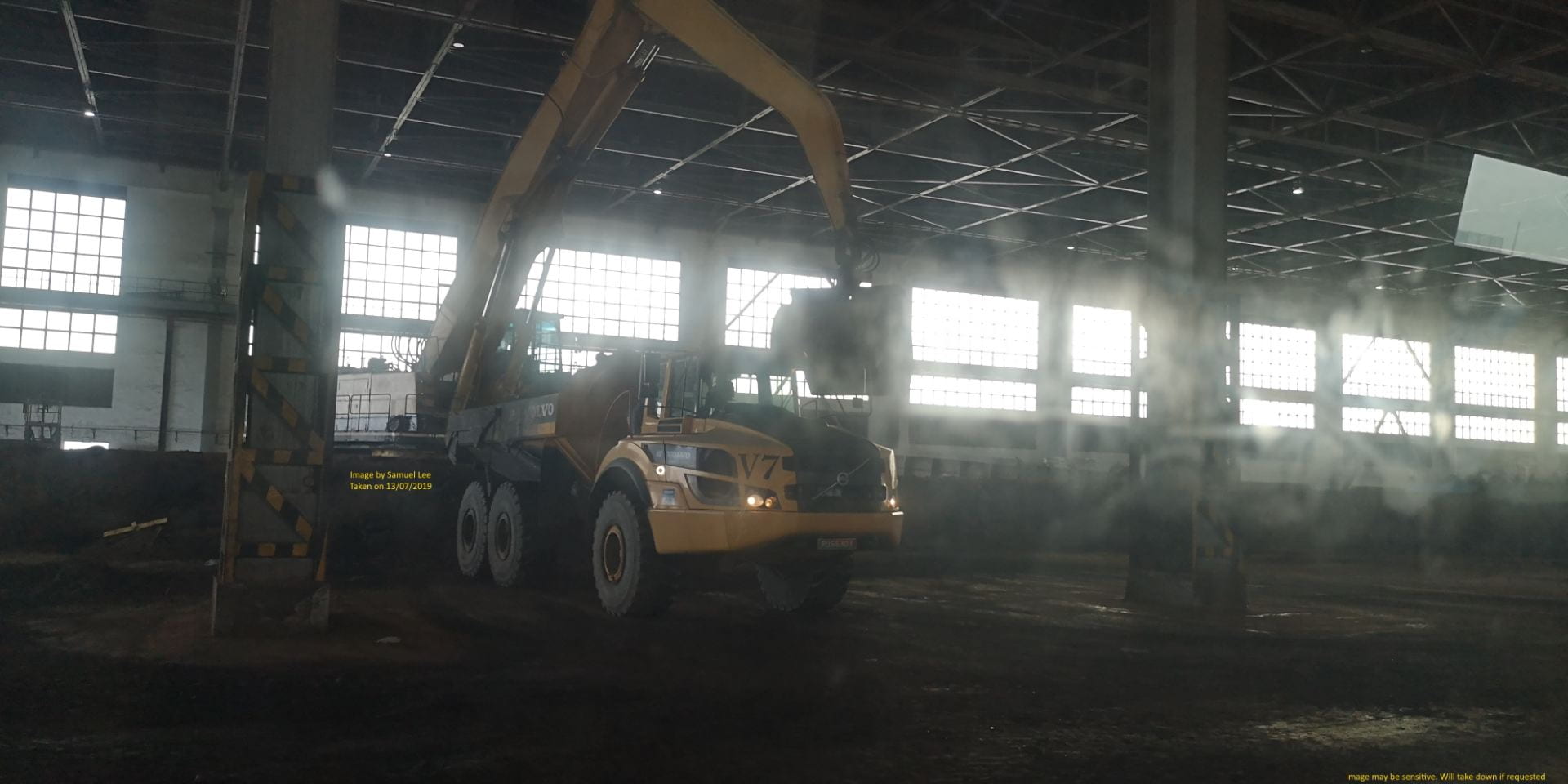
Bottom ash is carried by these dump trucks to the floating bottom ash dumping platform
Now at Phase II of development, BA is being dumped into a large enclosed lagoon as seen below.

Map of Semakau landfill. Full credit goes to NEA for the map.
This lagoon is enclosed by granite bungs with a layer of impermeable material extending all the way to the sea bed, seen here.
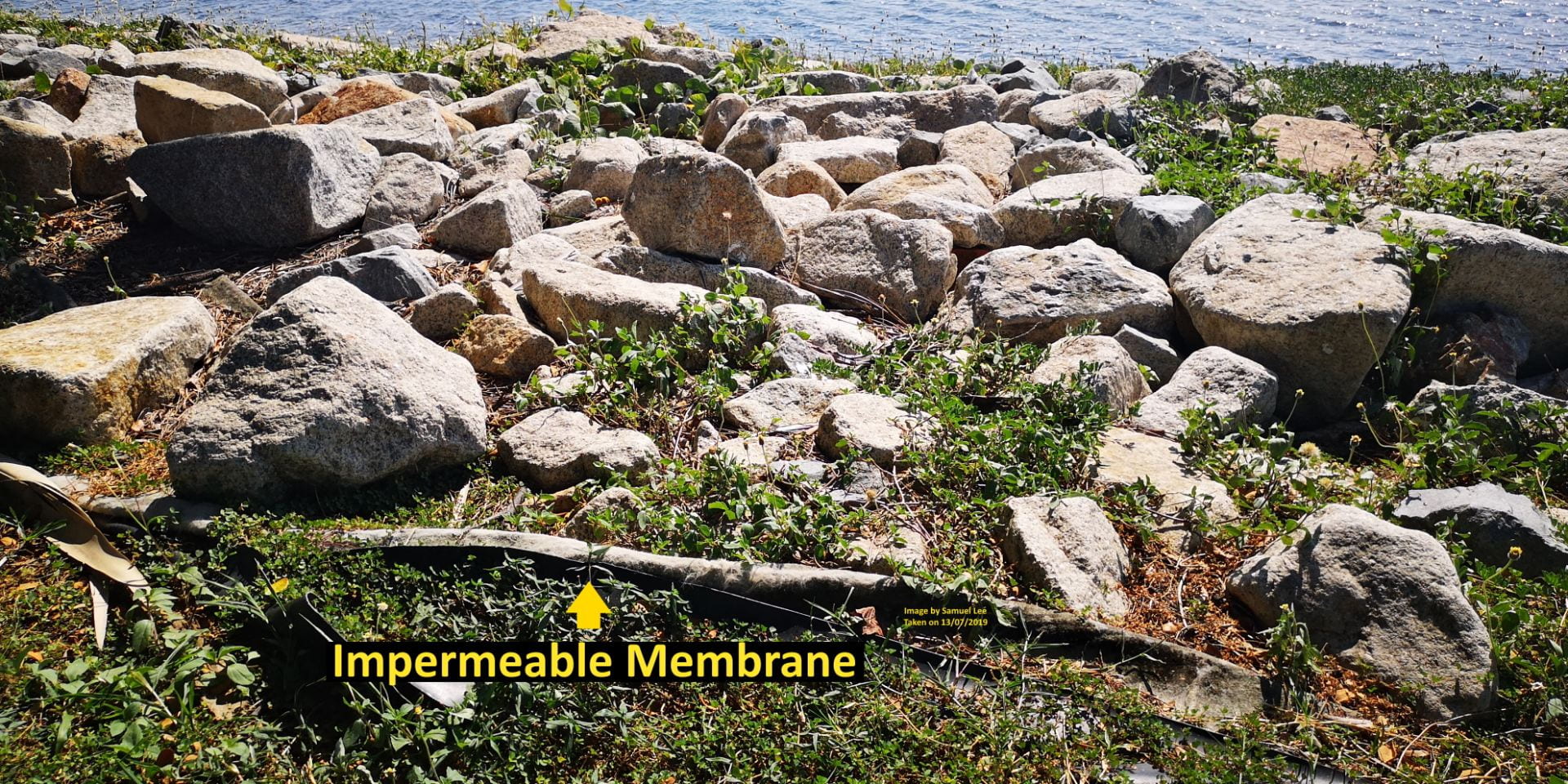
Granite bung with an impermeable membrane at Phase II lagoon
As more ash is filled in, the lagoon’s water level rises, necessitating overflow discharge into the sea. This discharge is treated by a floating WWTP such that it meets the allowable limits for trade effluent discharge into a watercourse‡. The floating WWTP can be seen in the distance below.

Mockup of Phase II lagoon showing the floating dumping platform (longer) and the floating WWTP (shorter). Full credit goes to NEA for the mockup.
I also found an intriguing infographic on the technical specifications of the WWTP:

Infographic on the Semakau WWTP. Full credit to Memiontec Pte Ltd. and NEA for the infographic
We can conclude from these observations that direct exposure of BA leachate to seawater is entirely prevented.
However, a particular remark by one of our guides struck me: that at the bottom of the lagoon is the original seabed. This would mean that years after Phase II has been filled, the BA could become highly acidic after being repeatedly exposed to haze, polluted air from nearby Shell-owned Pulau Bukom, and bacteria from topsoil used to reforest the Phase II site(Li, Ohtsubo, Higashi, Yamaoka, & Morishita, 2007); thus resulting in heavy metals possibly leaching into the seabed and the surrounding sea.
Also, while the infographic does substantially more information than what is available online, much of it is redundant. Being in the public lobby, one could assume the infographic is meant for the public. Personally, a technology showcase and a detailed characterisation of the Phase II lagoon water chemistry would have been more appropriate. Of course, this data is sensitive; but I do find it a missed opportunity to build trust with the public about the competency of local treatment facilities.
Sadly, when I tried to get in contact with Memiontec for an interview on these queries, I did not get a reply.
References:
Dung, T., Vassilieva, E., Swennen, R., & Cappuyns, V. (2018). Release of Trace Elements from Bottom Ash from Hazardous Waste Incinerators. Recycling, 3(3), 36. https://doi.org/10.3390/recycling3030036
Li, L. Y., Ohtsubo, M., Higashi, T., Yamaoka, S., & Morishita, T. (2007). Leachability of municipal solid waste ashes in simulated landfill conditions. Waste Management. https://doi.org/10.1016/j.wasman.2006.04.014
Lin, C. F., Wu, C. H., & Liu, Y. C. (2007). Long-term leaching test of incinerator bottom ash: Evaluation of Cu partition. Waste Management. https://doi.org/10.1016/j.wasman.2006.07.004
Manaia, C. M., Rocha, J., Scaccia, N., Marano, R., Radu, E., Biancullo, F., … Nunes, O. C. (2018). Antibiotic resistance in wastewater treatment plants: Tackling the black box. Environment International. https://doi.org/10.1016/j.envint.2018.03.044
PUB (2016) Sludge Dewatering Used Water Treatment Process. Retrieved from: https://www.pub.gov.sg/usedwater/treatment/usedwatertreatmentprocess
Tchounwou, P. B., Yedjou, C. G., Patlolla, A. K., & Sutton, D. J. (2012). Heavy metal toxicity and the environment. EXS. https://doi.org/10.1007/978-3-7643-8340-4_6
Additional Notes
* I got this piece of information from my NEA mentors during my internship in 2019, as well as through queries to NSL Oilchem Tuas 2’s site directors on a field trip.
† Incineration results in Fly Ash and Filter Cake that ends up in the flue gas filters too, but these are sent for further treatment before disposal on Pulau Semakau, according to an NEA guide on a Tuas Incineration Plant tour.
‡ This refers to Annex H of SS593 Code of Practice for Pollution Control on Page 42-43
One Man’s Cure is Everybody’s Poison
Last week, we discussed the problems with antibiotics and how municipal wastewater treatment plants(WWTP) like ours worsen the spread of antibiotics, antibiotic-resistant genes(ARG) and antibiotic-resistant bacteria(ARB) into the environment.
This week, we expand our scope on pollutants to the subset of WHO’s persistent organic pollutants that encompass antibiotics, biocidal compounds, pharmaceuticals and endocrine disruptors; which I’ll term as bio-disruptive organic compounds (BOC) for the purpose of this post.
BES folks might recognise endocrine disruptors as an emerging pollutant in freshwater systems; but for the sake of those unaware, these BOCs alter hormone levels in organisms in a way that causes harm to the organism, its offspring, or those around them(Bergman, Heindel, Jobling, Kidd, & Zoeller, 2012; Godfray et al., 2019).
As the term “emerging pollutant” might allude to, new endocrine disruptors and BOCs are being discovered or manufactured(Bergman et al., 2012). Some of these originate from completely unintuitive sources: I mean, imagine flame retardants changing your hormone levels(Godfray et al., 2019)! I wouldn’t even have thought to look there for BOCs!
Not to mention, household products (especially plastics, glues, lubricating oils) contain plasticizers like phthalates, BPA and flame retardants like Polybrominated diphenyl ethers(PBDE) that are also endocrine disruptors(Monneret, 2017).
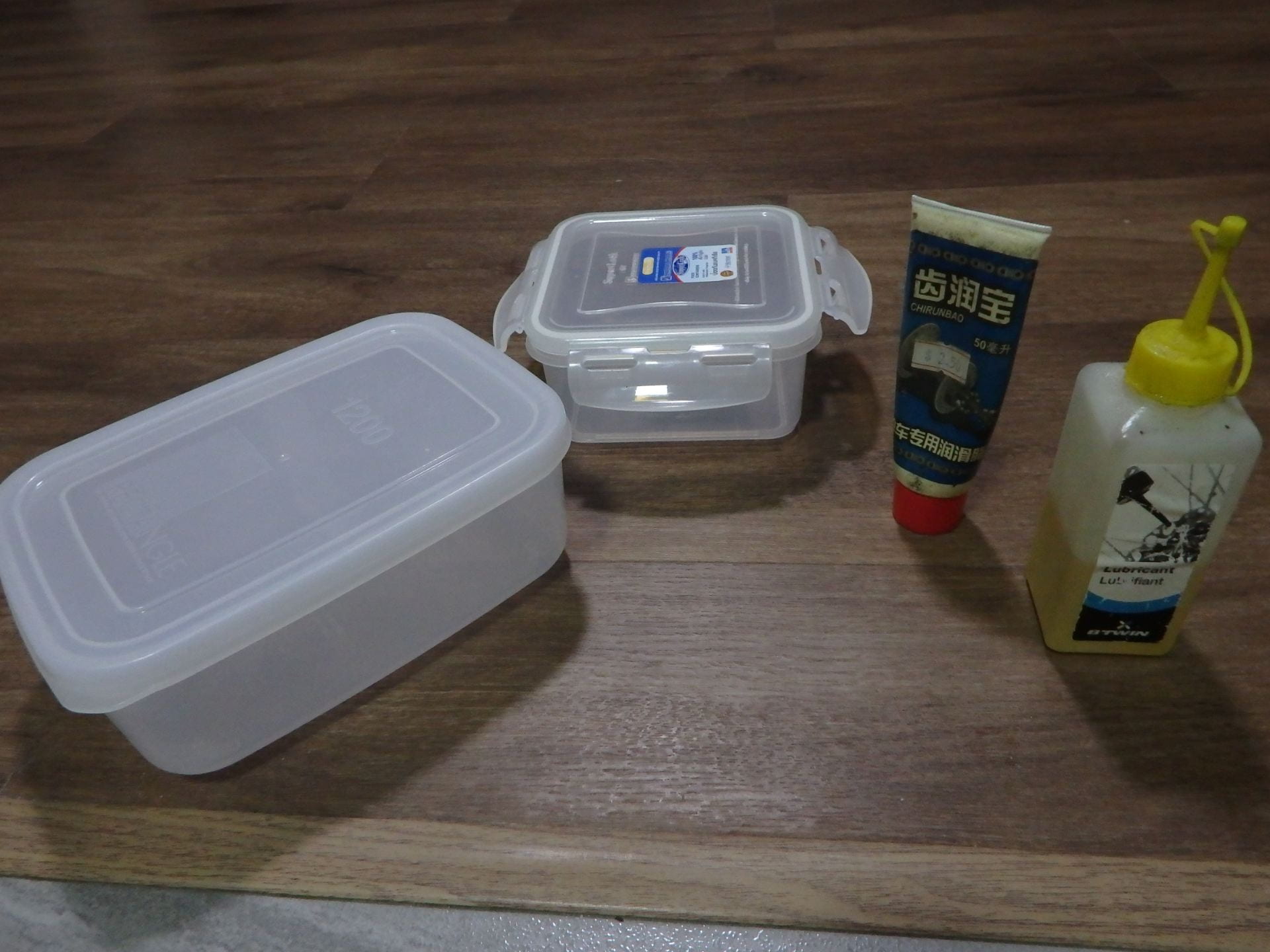
Yes…even I’m guilty.
Plastic containers, lubricants, and even vinyl flooring contain BOCs that can be washed into our sewers(Monneret, 2017).
Think about it, every time you wash your utensils, ride a public bus or wash your floor: we are washing BOCs down our sewers. With all due respect to PUB, our municipal WWTPs are still under-equipped to ensure complete and utter removal of all BOCs from our wastewater stream(Sui et al., 2015). I must stress utter removal because BOC concentrations of parts per trillion(ppt) are still harmful(Godfray et al., 2019).
Remember our 4 national taps? Yep, that same catchment water Sui et al (2015) found BOCs in bounces straight back at us. Of course, nothing is happening to our aquatic ecosystems or drinking water as of yet; but we can’t say with certainty that there will not be an ARB outbreak., an emergent BOC contamination, or any side effects of chronic exposure to BOCs
No point crying over spilt drugs, let’s talk solutions. The problem? Elevated concentrations of Diclofenac and Carbamazepine in ppt to ppb, and Caffeine in ppb to ppm; found around the Jurong Lake Catchment(Tran, Li, Hu, & Ong, 2014).
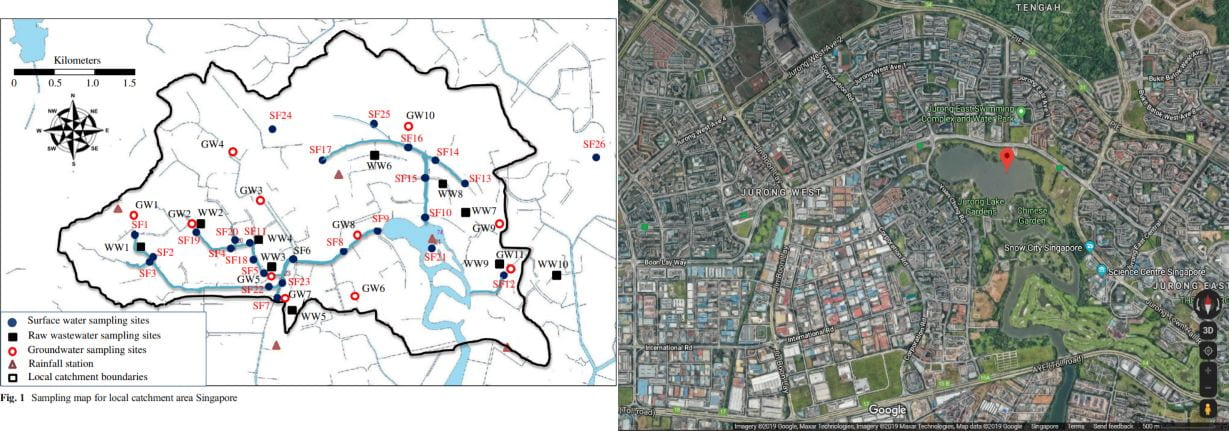
Sampling sites for pharmaceuticals around Jurong Lake Catchment.
Source(left): https://doi.org/10.1007/s11356-013-2428-9
Source(right): https://www.google.com/maps/place/Jurong+Lake/@1.3382125,103.7046754,4171m/data=!3m1!1e3!4m5!3m4!1s0x31da0ffd5cd73093:0x415a1e9ba09be19b!8m2!3d1.3418479!4d103.7281926
While there probably won’t be a universal solution to eliminate these BOCs from our wastewater, there is a particular pilot test that achieved significant results in a context analogous to Singapore’s WWTPs that I would like to present. A pilot plant utilising a novel technology, known as Membrane Distillation(MD), was implemented at Swedish WWTP as an alternative to an existing reverse osmosis array for the purpose of pharmaceutical wastewater treatment(Fortkamp et al., 2015). To put it simply, instead of using high pressures to squeeze water through a porous membrane, a selective hydrophilic membrane that only allows vaporised wastewater through is used.
The pilot managed to reduce Diclofenac, Carbamazepine, and Caffeine concentrations to below 3 ppt(Fortkamp et al., 2015). However, outstanding costs for heating wastewater was identified(Fortkamp et al., 2015). Fortunately, since PUB’s WWTPs recycles biogas for energy generation, a similar MD system with virtually no extra energy expenditure may be possible!
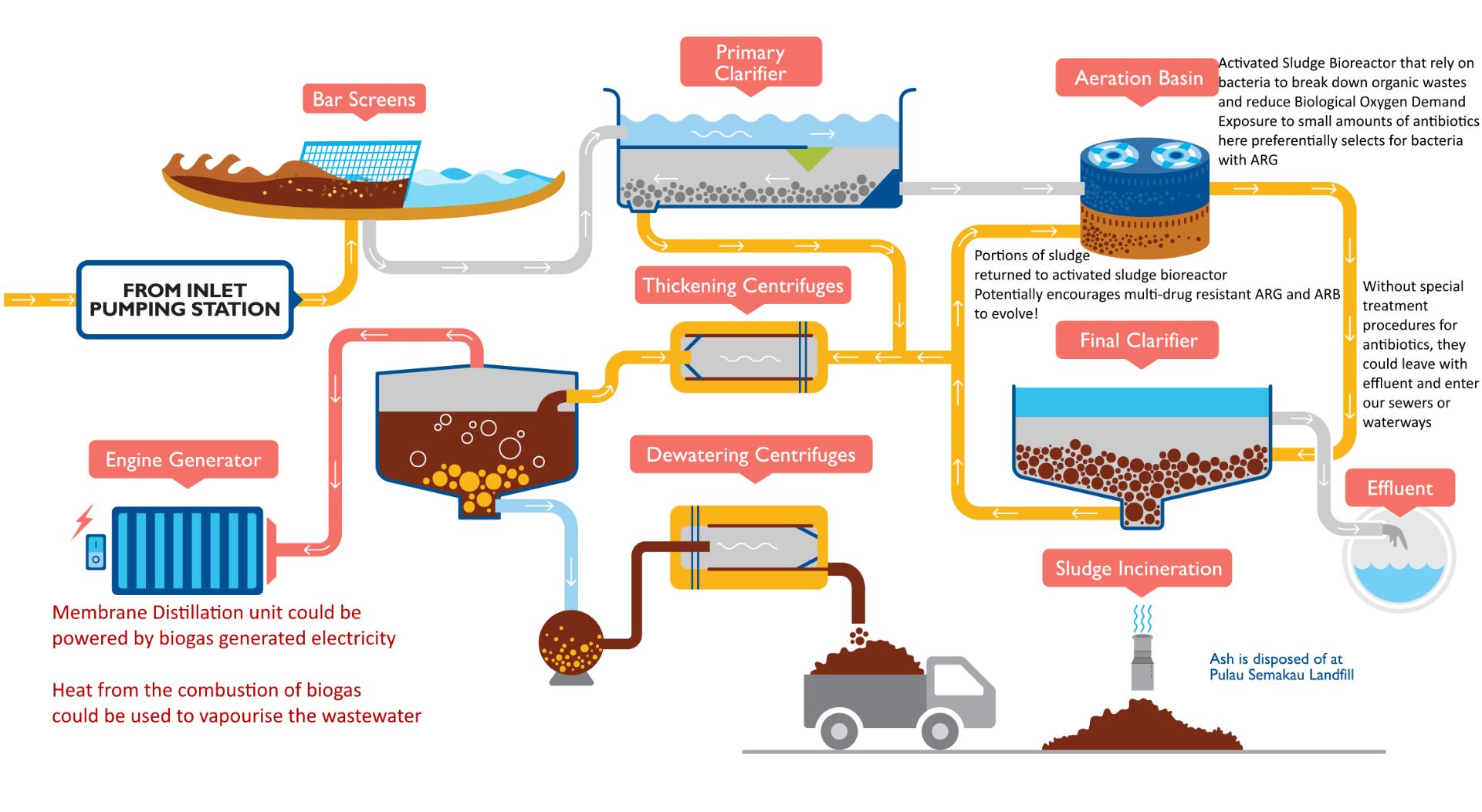
A possible opportunity to install a membrane distillation unit in our WWTPs. Source: https://www.pub.gov.sg/PublishingImages/PUB_29_UsedWaterConventionalTreatment%20PA-01.png
References:
Bergman, Å., Heindel, J., Jobling, S., Kidd, K., & Zoeller, R. T. (2012). State-of-the-science of endocrine disrupting chemicals, 2012. Toxicology Letters. https://doi.org/10.1016/j.toxlet.2012.03.020
Fortkamp, U., Royen, H., Klingspor, M., Ekengren, Ö., Martin, A., & Woldemariam, D. M. (2015). Membrane Distillation pilot tests for different wastewaters.In IVL Report B 2236: https://www.ivl.se/download/18.7e136029152c7d48c201f3/…/B2236.pdf
Godfray, H. C. J., Stephens, A. E. A., Jepson, P. D., Jobling, S., Johnson, A. C., Matthiessen, P., … McLean, A. R. (2019). A restatement of the natural science evidence base on the effects of endocrine disrupting chemicals on wildlife. Proceedings of the Royal Society B: Biological Sciences. https://doi.org/10.1098/rspb.2018.2416
Monneret, C. (2017). What is an endocrine disruptor? Comptes Rendus – Biologies. https://doi.org/10.1016/j.crvi.2017.07.004
Sui, Q., Cao, X., Lu, S., Zhao, W., Qiu, Z., & Yu, G. (2015). Occurrence, sources and fate of pharmaceuticals and personal care products in the groundwater: A review. Emerging Contaminants. https://doi.org/10.1016/j.emcon.2015.07.001
Tran, N. H., Li, J., Hu, J., & Ong, S. L. (2014). Occurrence and suitability of pharmaceuticals and personal care products as molecular markers for raw wastewater contamination in surface water and groundwater. Environmental Science and Pollution Research. https://doi.org/10.1007/s11356-013-2428-9
Additional Note:
If you would like to read more about Membrane Distillation, check out this book at National Libraries!
Specifically, pages 305-350. Really interesting stuff!
A Failing Panacea
From radioactive sea monsters to mutant superbugs. This week, I’ll be discussing antibiotic-resistant viruses and their relationship with wastewater treatment!
Antibiotic compounds have flooded and permeated into every nook and cranny of our society. They are commercially utilized for livestock and aquaculture to prevent diseases outbreaks; applied to crops to improve disease resistance and increase yields; and even prescribed by clinics and hospitals(Kümmerer, 2009) for minor ailments like giving candy to children during Halloween!
Antibiotic introduction into the natural environment come from both non-point sources (surface run-off, groundwater permeation etc.) and this post’s primary focus: point source from wastewater treatment plants(WWTP) and direct discharge(Kümmerer, 2009). However, the real danger does not arise from the antibiotics released itself, but what happens when bacteria and viruses present and utilized in biochemical treatment in WWTPs receive a sublethal dose of antibiotics(Manaia et al., 2018).
That’s right! For the same reason your doctor tells you to finish the prescribed dose of antibiotics, a non-lethal dose of antibiotics combined with bioreactor conditions that boost bacterial growth hosts a favourable environment for bacteria with antibiotic-resistant genes(ARG) to outcompete their regular counterparts(Manaia et al., 2018).

Potential for ARG and ARB to develop in PUB’s wastewater treatment system. Source: https://www.pub.gov.sg/PublishingImages/PUB_29_UsedWaterConventionalTreatment%20PA-01.png
To make things worse, many bioreactors (like in PUB’s municipal WWTPs) recycles portions of bacterial sludge from previous batches, exposing the antibiotic-resistant bacteria(ARB) to a wide variety of other antibiotics(Manaia et al., 2018). These fluctuating conditions preferentially select bacteria and viruses with ARG that resist a wide variety of antibiotics(Manaia et al., 2018), similar to Dr Rick Pott’s Variability Selection hypothesis.
If you’ve read A Clearer Picture, you might be wondering: since the wastewater ends up being disinfected before being discharged, why worry? While most ARB are vulnerable to disinfection, some studies show that ARG go through WWTPs largely untouched where they may be transferred to other cells in the natural environment(Yuan, Guo, & Yang, 2015); some ARB can even go into dormancy only to be reactivated once out of the disinfection tank(Manaia et al., 2018). Of course, antibiotics also make it out of the treatment process(Kümmerer, 2009), which makes it easier for bacteria in natural ecosystems to evolve into ARBs.
The consequences of this inadequacy are all around us. Wei et al. (2018) found significant amounts of ARG in bioreactor bacteria in Chinese WWTPs; Hatosy & Martiny (2015) found known and undiscovered ARG in coastal waters of California and Hawaii; and to top it all off, two separate studies found ARBs in bottlenose dolphins, harbour seals and harbour porpoises ((Schaefer et al., 2019), and this preliminary study conducted in Puget Sound).
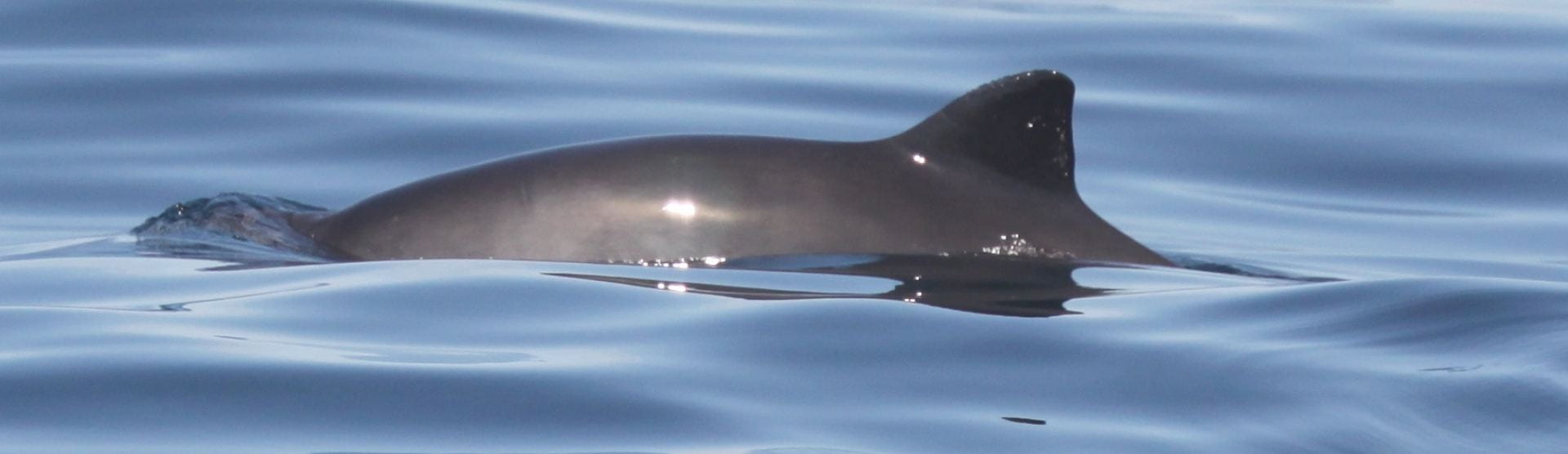
A Harbour Porpoise, one of the hosts of ARB found in a preliminary study. Harbour Porpoise ©Niki Clear. Source: https://www.wildlifetrusts.org/wildlife-explorer/marine/marine-mammals-and-sea-turtles/harbour-porpoise
All that is left for a potentially apocalyptic outbreak is for the ARG to incorporate itself in a human vector or pathogen(like E. Coli) or god forbid, an influenza virus with multiple ARGs that resists all kinds of antibiotics, a superbug(Manaia, 2017).

Possible Pathways for ARG and ARB to make their way from natural systems into human and livestock. Source: (Manaia et al., 2017)
If it isn’t clear enough already, excessive antibiotic use not the way forward. With ARG all over our biosphere, it’s just a matter of time before a superbug pandemic matching the scale of the black plague starts. Antibiotic substitutes like Yan Yang et al.’s polymer could be our salvation. Until then, go organic, eat fewer products that use antibiotics(like meat and dairy); and please, finish your antibiotic dose. Doctor’s orders!
Next week, I’ll be looking at the ways to deal with antibiotics and pharmaceuticals right at the source, so stay tuned!
References:
Hatosy, S. M., & Martiny, A. C. (2015). The ocean as a global reservoir of antibiotic resistance genes. Applied and Environmental Microbiology. https://doi.org/10.1128/AEM.00736-15
Kümmerer, K. (2009). Antibiotics in the aquatic environment – A review – Part I. Chemosphere. https://doi.org/10.1016/j.chemosphere.2008.11.086
Manaia, C. M. (2017). Assessing the Risk of Antibiotic Resistance Transmission from the Environment to Humans: Non-Direct Proportionality between Abundance and Risk. Trends in Microbiology. https://doi.org/10.1016/j.tim.2016.11.014
Manaia, C. M., Rocha, J., Scaccia, N., Marano, R., Radu, E., Biancullo, F., … Nunes, O. C. (2018). Antibiotic resistance in wastewater treatment plants: Tackling the black box. Environment International. https://doi.org/10.1016/j.envint.2018.03.044
Schaefer, A. M., Bossart, G. D., Harrington, T., Fair, P. A., McCarthy, P. J., & Reif, J. S. (2019). Temporal Changes in Antibiotic Resistance Among Bacteria Isolated from Common Bottlenose Dolphins (Tursiops truncatus) in the Indian River Lagoon, Florida, 2003-2015. Aquatic Mammals, 45(5), 533–542. https://doi.org/10.1578/AM.45.5.2019.533
Wei, Z., Feng, K., Li, S., Zhang, Y., Chen, H., Yin, H., … Deng, Y. (2018). Exploring abundance, diversity and variation of a widespread antibiotic resistance gene in wastewater treatment plants. Environment International. https://doi.org/10.1016/j.envint.2018.05.009
Yuan, Q. Bin, Guo, M. T., & Yang, J. (2015). Fate of antibiotic resistant bacteria and genes during wastewater chlorination: Implication for antibiotic resistance control. PLoS ONE. https://doi.org/10.1371/journal.pone.0119403
60,000Bq/L… Not Great, Not Terrible
Wow, this just popped out of the blue. Japan’s environmental minister stated in a press release that Tokyo Electric Power(TEPco) will have to dump Fukushima nuclear powerplant’s radioactive wastewater into the ocean for dilution as it runs out of storage space (one of the many articles). Well doesn’t that sound like the plot to 2014’s Godzilla!
Irradiated sea monsters aside, there are serious controversies and environmental implications of this decision. The Korean government and Japanese fishermen are quite understandably vocal about their concerns with this decision (McCurry, 2019). Meanwhile, Osaka offers to dump radioactive wastewater into Osaka bay as long as it only contains tritium and is environmentally safe (Johnston, 2019).
What’s the big issue with dumping radioactive wastewater? Radionuclides! Specifically, Caesium-134(134Cs), Caesium-137(137Cs), Strontium-90(90Sr), and Tritium(3H), the most prevalent nuclear fallout products at Fukushima now(TEPco, 2019). These radionuclides emit high-energy particles when they decay, which literally tears up DNA upon contact. This causes cells to reproduce into defunct and cancerous cells or even cell death(S. Dingwall, C.E. Mills, N. Phan, K. Taylor, 2011; Starr; Taggart; Evers; Starr, 2019).
Water treatment at Fukushima Daiichi nuclear plant involves treating the water used to cool the damaged fuel rods in Reactors 1, 2, and 3. The main processes are:
- Ion-exchange modules (Kurion/Sarry) that exchange strontium and caesium ions for less harmful ones.
- Reverse osmosis (RO) that recycles clean water into the reactor core and concentrates remaining brine
- Adsorption of remaining caesium, strontium and other (heavy) metals onto various substrates

Treatment facility for processes 1 and 2. Source: https://www.meti.go.jp/english/earthquake/nuclear/decommissioning/pdf/20190725_e.pdf
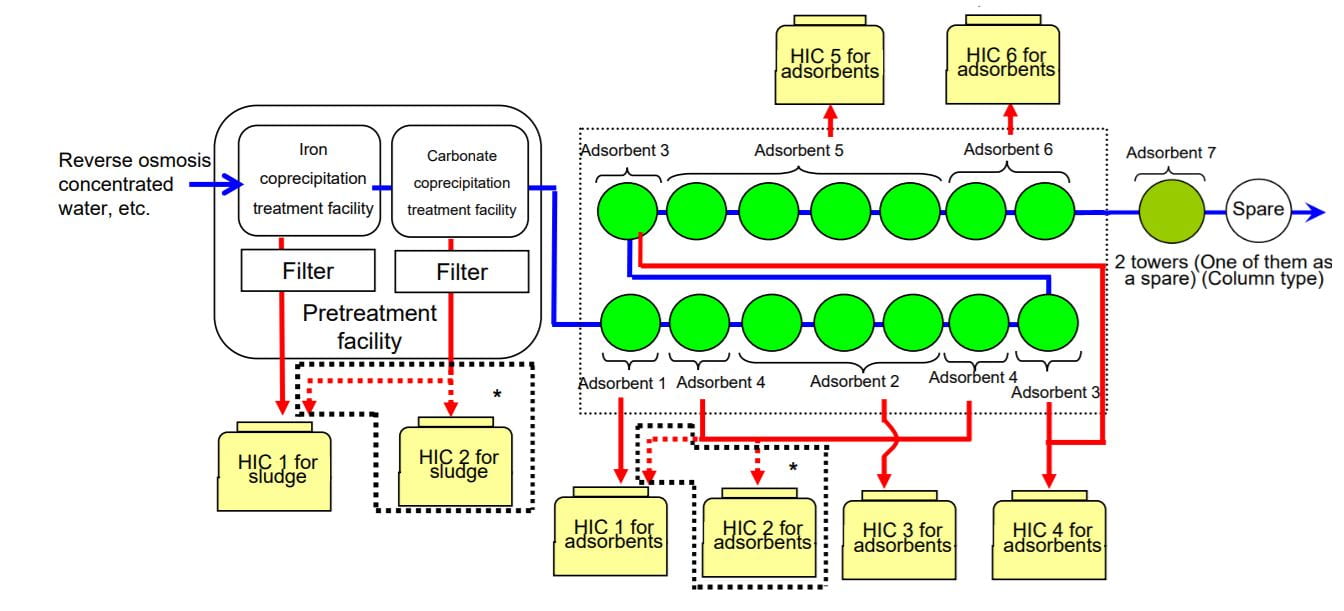
“Multi-nuclide removal equipment” for process 3. Source: https://www7.tepco.co.jp/wp-content/uploads/hd03-02-03-001-m120625_01-e.pdf

Table of Adsorptive Substrates used. Source: https://www7.tepco.co.jp/wp-content/uploads/hd03-02-03-001-m120625_01-e.pdf
Now you might have noticed that there is a radionuclide I listed earlier that is missing from the treatment process above. That’s right, it’s tritium! Being an isotope of hydrogen, it is incorporated into a H2O molecule as HTO, and passes seamlessly through all of the processes above just like plain old water. Yet, HTO is as dangerous as any radionuclide when tritium decays. What’s worse, TEPco has 800,000m3 of tritiated water sitting around with no feasible isotopic separation method (METI, 2016).
Fortunately, tritium ionizing radiation possesses very little energy and is unable to pass through physical environments or even the layer of dead skin on your epidermis. Contamination is therefore primarily through ingestion or inhalation of tritiated molecules. Dingwall et al. (2011) referred to Brooks et al. (1976) that found that mice could receive 37,000,000 Bq/L of tritiated water without damaging their DNA and causing adverse health effects.

Source: https://www.meti.go.jp/english/earthquake/nuclear/decommissioning/pdf/20190801_01a.pdf, https://www.meti.go.jp/english/earthquake/nuclear/decommissioning/pdf/20190801_01b.pdf
The Tritiated Water Task Force of TEPco estimates a tritiated water concentration of 0.3 to 3.3million Bq/L as of March 2016. They plan to dilute the tritiated water with seawater until concentration levels are 60,000Bq/L before discharge. They also estimate this concentration to drop by 3 orders of magnitude 100km away from the point of discharge (ie.a maximum of 60 Bq/L). To provide perspective, this exceeds most standards of tritium in drinking water.
With all the bad press surrounding Japan, we need to recognise that environments close to the source of discharge face potentially unknown effects of concentrated tritiated water (IRSN, 2012); as well as acknowledge what they have accomplished to reduce impacts as much as they have. Less fear-mongering, more research!
References:
Brooks, A. L., Carsten, A. L., Mead, D. K., Retherford, J. C., & Crain, C. R. (1976). The Effect of Continuous Intake of Tritiated Water (HTO) on the Liver Chromosomes of Mice. Radiation Research. https://doi.org/10.2307/3574329
Cecie Starr; Ralph Taggart; Christine Evers; Lisa Starr. (2019). Biology: The Unity and Diversity of Life, 15th Edition. Retrieved from https://www.cengage.com/c/biology-the-unity-and-diversity-of-life-15e-starr/
Dingwall, S., Mills, C. E., Phan, N., Taylor, K., & Boreham, D. R. (2011). Human health and the biological effects of tritium in drinking water: Prudent policy through science – Addressing the ODWAC new recommendation. Dose-Response. https://doi.org/10.2203/dose-response.10-048.Boreham
E. Johnston (2019). Osaka mayor Ichiro Matsui offers to take in tainted Fukushima water and dump it into Osaka Bay. Japan Times. https://www.japantimes.co.jp/news/2019/09/17/national/osaka-mayor-offers-take-tainted-fukushima-water-dump-osaka-bay/#.XYMScCgzZEY
IRSN (2012) Radionuclide sheet: Tritium and the environment. Institut de radioprotection et de sûreté nucléaire. https://www.irsn.fr/EN/Research/publications-documentation/radionuclides-sheets/environment/Pages/Tritium-environment.aspx
J. McCurry (2019). Fukushima: Japan will have to dump radioactive water into Pacific, minister says. The Guardian. https://www.theguardian.com/environment/2019/sep/10/fukushima-japan-will-have-to-dump-radioactive-water-into-pacific-minister-says
METI (2016).Tritiated Water Task Force Report, June 2016. Ministry of Economy, Trade and Industry https://www.meti.go.jp/english/earthquake/nuclear/decommissioning/pdf/20160915_01a.pdf
Appendix:

Radionuclide concentrations around reactor sites(pardon my underlining skills)
Source for Table: https://www4.tepco.co.jp/decommission/data/analysis/pdf_csv/2019/3q/2tb-east_19091301-j.pdf
Source for sampling sites around the reactors: https://www4.tepco.co.jp/en/nu/fukushima-np/f1/smp/form_pdf/2tb-east_form-e.pdf
A Clearer Picture
Since my last post dealt with the importance of water treatment in Singapore, I think it’ll be quite fitting to make this one about the process of water treatment. PUB actually has rather well designed and concise infographics that introduces their water treatment system (check this and this out for more information). Therefore I’ll add on some details they may have missed out on, and also tap on my personal knowledge of the treatment techniques used by Toxic Industrial Waste Collectors (TIWCs) in Singapore from my internship.
Let’s try a little exercise! Head to Google Maps and look up satellite images of PUB’s water reclamation plant in Singapore. What caught your eye?
Was it these structures? I sure hope so! These are the core part of most water treatment plants, the bioreactors and clarifiers for settling bacteria aggregates after biochemical treatment.
These are like giant beer barrels; except instead of yeast, the bacteria cultures used are designed to digest and break down a wide variety of polysaccharides, lipids, and other pollutants.
They are the workhorse of any water treatment facility and help cut BOD and COD of wastewater down to safe levels for human use. The other processes of a water treatment plant revolve around the welfare and performance of these little guys.
The bacteria require high surface area access to pollutants, but high TSS and settleable solids just get in their way. Therefore, plants incorporate filter screens to remove large settleable solids and chemical coagulation and clarification of TSS.
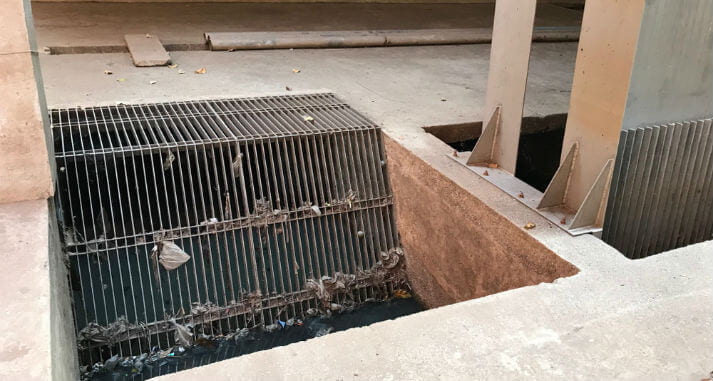
Bar screen for filtering large settleable solids. Source: https://aosts.com/types-wastewater-screening/
Saline wastewater reducing the productivity of bioreactor? (Linaric et al., 2013) Then pass the wastewater through a distillation set up to reduce TDS through evaporation!

A multiple-effect(stage) Distillation set up for lowering TDS Source: https://gemina.es/files/catalogue/pdf/18_Evaporadores_ING.pdf
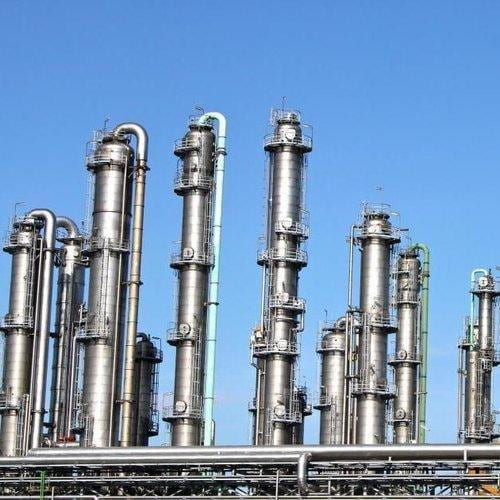
Multiple-effect distillation columns Source: https://www.indiamart.com/proddetail/distillation-column-21227565788.html
Heavy metals slowing down the growth of bacteria? (Cabrero et al., 1998) One of our TIWCs implemented an Electric Coagulator that precipitates charged aqueous metal ions as solid metal particles out for filtration without the use of chemicals!
Refractory non-biodegradable VOC and SVOC like tetrahydrofuran slowing down or even sterilising bioreactors? (Yao et al., 2012) Actually, that’s one of the headaches plaguing water treatment in Singapore at the moment! Fortunately, novel technologies are being experimented and pilot tested by PUB and a few TIWCs. I’ll be covering this in a later post.
After the bioreactors have done their job, its time to add final touches to the water. This depends on what the water is being used for. Domestic and industrial potable use calls for pH balancing and sterilisation. Specialised industries that need ultra-pure water go to NEWater, where our crème de la crème of water treatment technologies resides.
Every step along the way is filled with its own intricacies and face their own set of problems that probably deserve articles of their own. Plenty of research is being done along every step, and novel technologies are being thrown around left and right. However, a healthy dose of scepticism due to economic feasibility, performance, and stringent policies blocks the path of innovation. Let’s hope it does not come down to environmental degradation and human death to get things moving.
Gallery

Satellite Images of Kranji(Top) and Jurong(Bottom) Water Reclamation Plant. Source: Google maps

Close-up of a clarifier for settling bacteria aggregates after biological treatment. Source: https://www.pub.gov.sg/usedwater/treatment/usedwatertreatmentprocess

Aerated Activated Sludge Tank. Source: https://www.watertechonline.com/sludge-treatment-efficiency/
References
Cabrero, A., Fernandez, S., Mirada, F., & Garcia, J. (1998). Effects of copper and zinc on the activated sludge bacteria growth kinetics. Water Research. https://doi.org/10.1016/S0043-1354(97)00366-7
Linaric, M., Markic, M., & Sipos, L. (2013). High salinity wastewater treatment. Water Science and Technology. https://doi.org/10.2166/wst.2013.376
Yao, Y., Lu, Z., Min, H., Gao, H., & Zhu, F. (2012). The effect of tetrahydrofuran on the enzymatic activity and microbial community in activated sludge from a sequencing batch reactor. Ecotoxicology, 21(1), 56–65. https://doi.org/10.1007/s10646-011-0765-3